The Nervous System Evolved
The nervous system is the primary means by which cells in the body communicate with one another. The endocrine system is adjacent to this, but is not synonymous—though it’s not going to be covered anywhere here.
By using the nervous system, different parts of an organism can coordinate to perform intricate tasks. Systems vary in complexity from simple cell-to-cell communication, which is arguably not a true “system”, to vast networks of specialized nerve cells, each with its own dedicated purpose.
In short, it allows different areas of the body to collaborate for tasks such as movement and thought.
Porifera, known colloquially as the sea sponges, are composed of a diverse phylum of aquatic animals. They are also the host of the simplest form of nervous communication: cell-to-cell, sometimes called intercellular signalling.
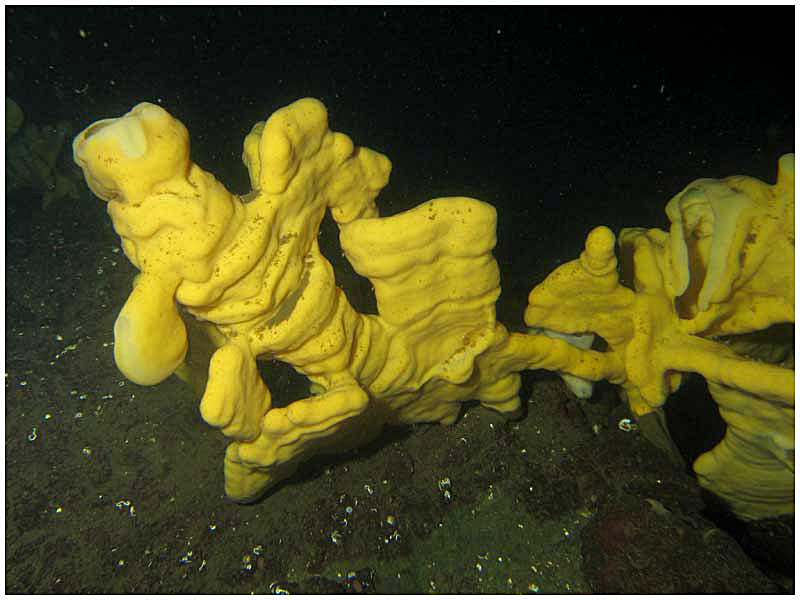
An example of Porifera. "Aphrocallistes vastus" | by Dan Hershman from Wikimedia Commons | Licensed under CC BY-SA 2.0
Cell-to-Cell Communication
Cell-to-cell is not typically considered to be a nervous "system", as there is no level of organization to it, but rather a nervous function. This function is not unique to Porifera, but they are unique in being the only animals whose cells send signals by this method alone.
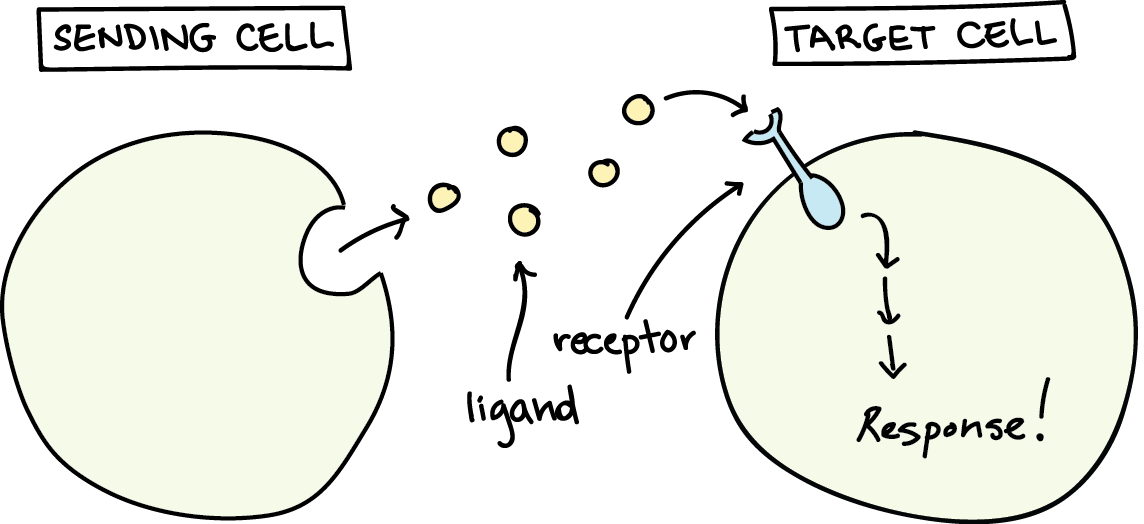
A diagram of cell-to-cell type communication.
With intercellular signalling, there are sensory proteins that penetrate the cell membrane and come into contact with other cells. There are also proteins on the inside of cells that detect chemicals, such as ligands, almost like a rudimentary endocrine system. These signals provide valuable insights, including information about water current activity, light levels, and what types of cells are nearby.
Even an apparently simple, crude function like intercellular signaling is essential in allowing eukaryotic organisms to coordinate actions.

Four different examples of the phylum Cnidaria. From top-left to bottom-right: a jellyfish, a soft coral, a rocky coral, and a sea anenome. By Frédéric Ducarme from Wikimedia Commons | Licensed under CC BY-SA 4.0
While the sponges were taking big evolutionary steps with its cell-to-cell communication, the phylum Cnidaria had gone a step further and formed the first dedicated nerve cells. While the "nerve net" of the Cnidaria's jellyfish and corals was not any more a 'system' than the signalling in Porifera, it was still an incredible stepping stone towards building the intricate nervous system us humans have.
Nerve Net
Again, this system was incredibly simple, but it contained the basic cell structure found in more complex nervous systems: the nerve cell. These specialized cells made intercellular communication more efficient and consistent than with cell-to-cell. In Porifera, chemical signals could be sent, but there was no guarantee that the signals would get to the right cells, or even be picked up by another cell at all. It was pivotal, yet faulty. The nerve net, on the other hand, acted like a set of telephone poles and wiring—albeit with a random distribution.

A nerve cell diagram with each part labelled. Source Bekolay, Trevor.
Nerve cells are made up of three parts: the soma, the axon, and the dendrite. The soma is the main part of the nerve cell; the base cell onto which the nervous equipment is mounted. It holds the cell's organelles and DNA, as well as other structures adapted for basic cell function and survival. The other parts allow the cell to transmit communicative electrical signals, the axon transmitting signals and the dendrite recieving them.
The nerve net was an important advancement for the nervous systems of all other animals, but especially to Cnidaria because they were moving away from the filter feeding lifestyle of sponges. These animals now required coordinated movement in order to hunt the microscopic planktonic organisms that were their prey.
They were able to achieve this with nerve cells and did not develop their nervous systems further like some other animal groups did.
Worms were the first animals to evolve a true central nervous system. There are three phyla of animals we traditionally call "worms": Platyhelminthes, Nematoda, and Annelida.
Platyhelminthes: Flatworms
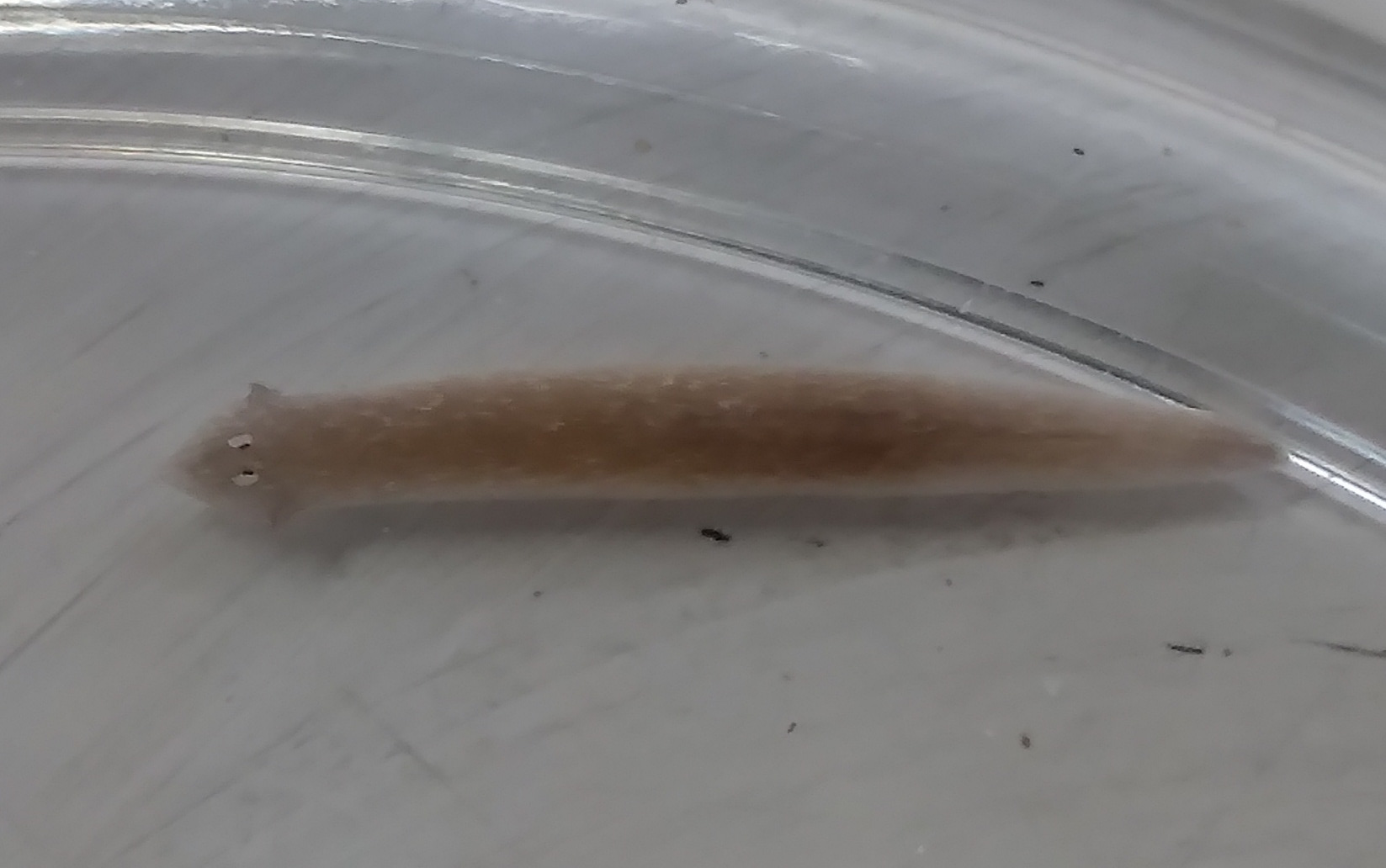
A planaria, a type of common, non-parasitic flatworm, in a petri dish filled with aquarium water. Image by me.
The simplest group of worms, the flatworm, is characterized by a large nerve cell mass in its head. This mass, called the ganglion, is divided into a top arch and two lumps of nerves called ganglia beneath the organism's eyes. Yes, this is also the first animal with complex eyes.
Flatworms, such as the planaria above, also have a pair of large sensory organs on their head. Called the auricles, they appear as triangular flaps on either side of the animal, and the sensitive skin allows the worm to feel where it's going. These worms also have two sets of muscles, a feature that the first two animal groups lacked.
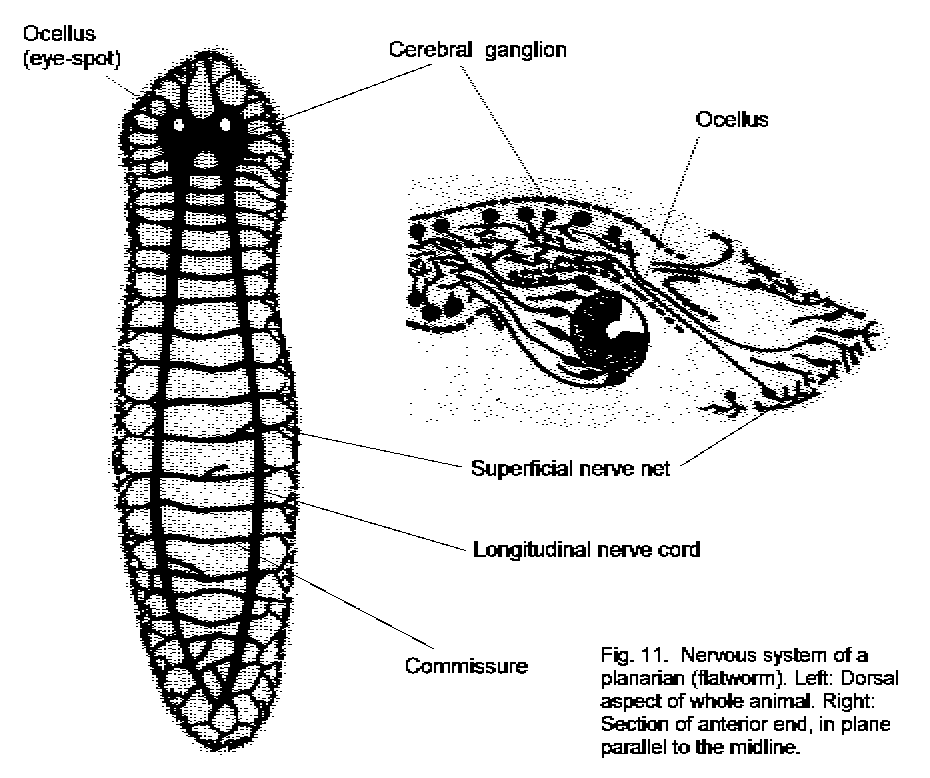
Planarian nervous system diagram.
The flatworm peripheral nerve structure appears in a ladder shape, with two nerve cords running down either side of the body connected at several points by nerve "rungs". The shape of the nervous system allowed early flatworms to move in a much more controlled, purposeful manor than the animals before it. The centralized ganglion of the worm right next to its major sensory organs allowed it to process sensory input and supposedly to have basic thoughts.
These advancements allowed planaria (as well as other flatworm species) to become a more capable predator that could actively and nomadically hunt its prey.
Nematoda: Roundworms
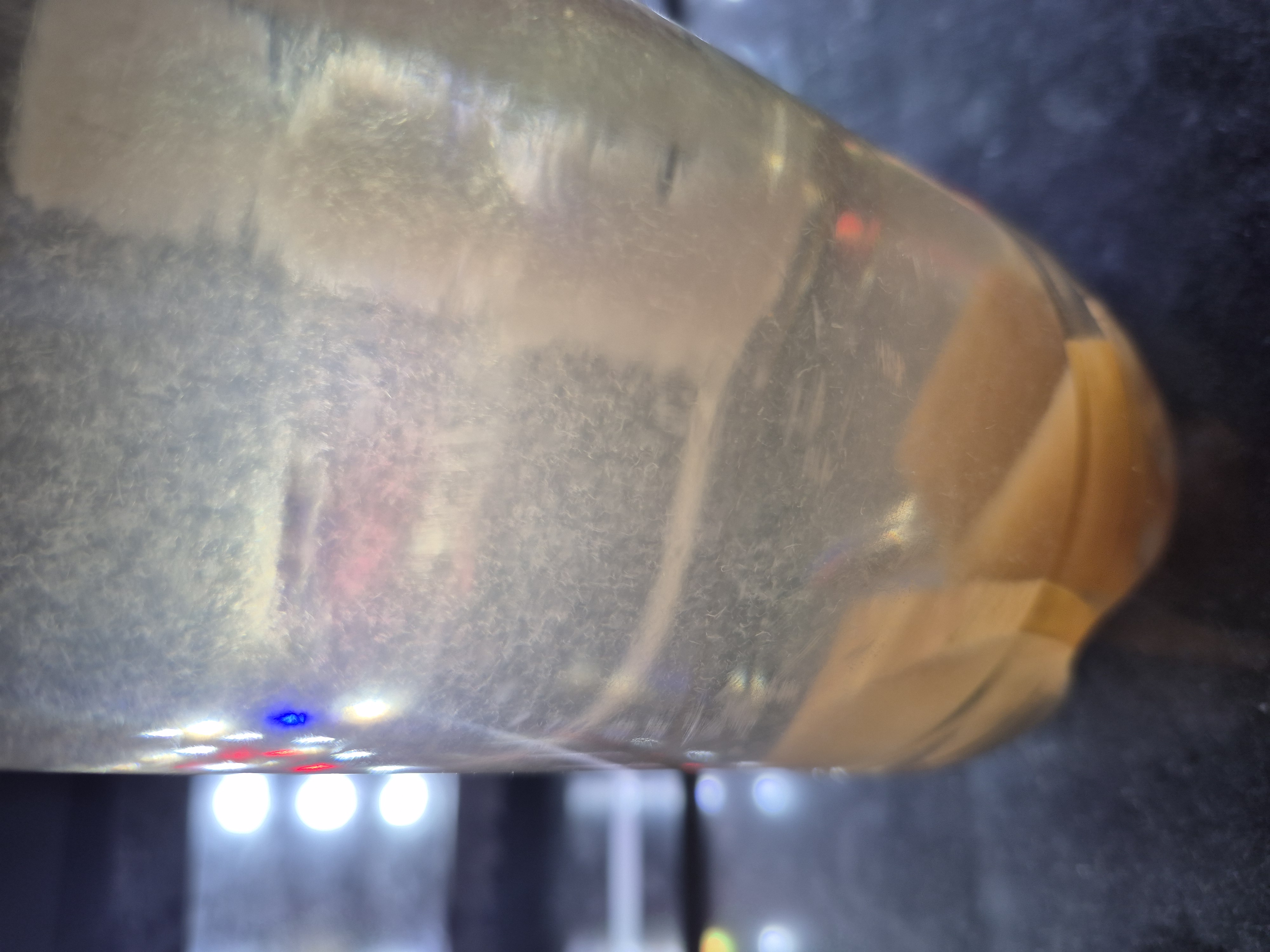
Vinegar eel culture in a soda bottle filled with 50% vinegar & 50% tap water. The apple slices are their food. Image by me.
In Nematoda, there is one less muscle set but four nerve cords—two lateral, a dorsal, and a ventral cord—in the nerve ladder instead of two. The rungs of the ladder connect to make multiple circular shapes. This nerve ring structure is useful to roundworms because it allows them to move more precisely despite having few muscles than the flatworms.
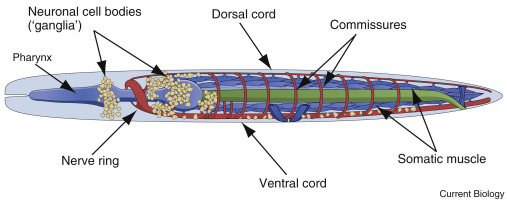
Roundworm nervous system diagram with lateral cords omitted for clarity. Source Schafer, William.
They also have a somewhat larger ganglion compared to the flatworms. They still don't have a true "brain", though.
Annelida: Segmented Worms
Annelids, composed of earthworms, leeches, and some oceanic worms, are a much more familiar group than Platyheminthes and Nematoda. They also have some unique nervous advancements that set them apart biologically from the other two worm phyla.
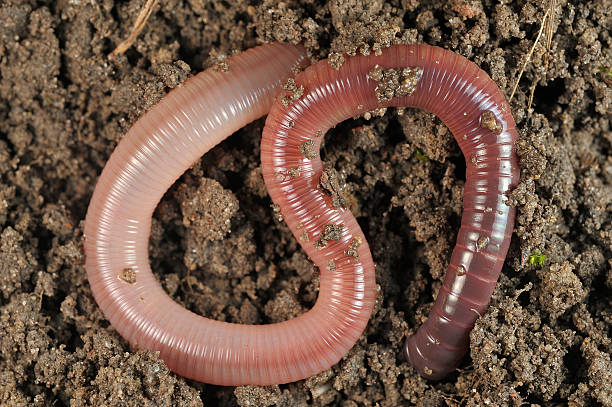
An earthworm. Note the creases on its skin, which make visible segments in worm's body. Stock image.
They are the first animals to have a true brain, composed of two nerve clusters—one on the right side of the body and one for the left. They have a brain stem that connects to a bodily nerve cord, similar to the other worms. However, instead of the nerve ring structure evenly spaced across the body, the rungs on their ladder are nerve masses in each divided segment of their body. These are called segmental ganglia, and they control muscles and organs in each segment.
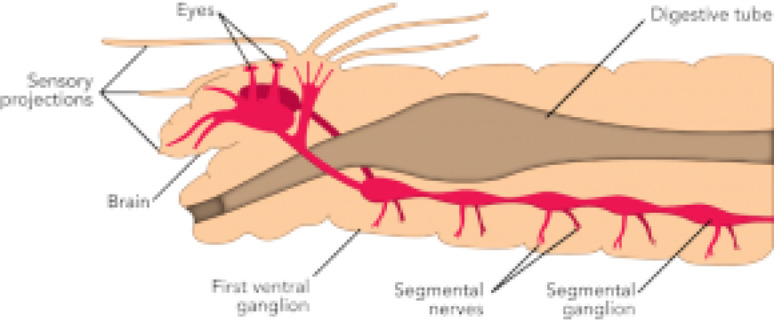
Nervous system of an oceanic annelid worm. Image by Byron Inouye.
Since there is a nerve cluster controlling each segment of the body, the pressure of determining small movements in the body is taken away from the brain, which is more free to perform cognitive tasks. Using their new relative intelligence allows earthworms to find food under the soil and escape their predators more consistently.
Also, while earthworms are eyeless, some sea worms have complex eyes that can distinguish shapes. This most likely allows them to hunt better.
Mollusks: Octopi
Mollusks are a diverse group containing animals from the simple slug to the elegant nautilus to the cyclopean squid. But, for the sake of simplicity and because the mollusk nervous system is variable even at the species level, I am only going to cover those in Order Octopi.
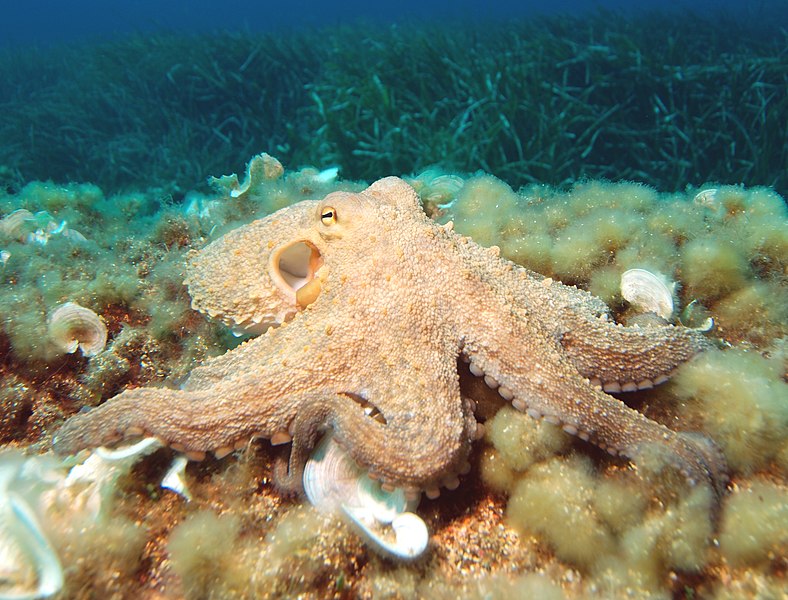
Photo of a common octopus, "Octopus vulgaris". Image by Albert Kok from Wikimedia Commons | Licensed under CC 3.0 by-SA
Like roundworms, octopi have nerve rings, but they also each have a brain like annelids do. Actually, they kind of have nine brains. While the central anterior brain is the largest, octopi have large nerve clusters—upwards of 40 million nerves—in each arm. Studies have also shown that these brains operate mostly independently from the main brain, their arms able to continue moving as they normally would even when severed from their body. These strange creatures have the largest nervous systems of any non-chordate animal.
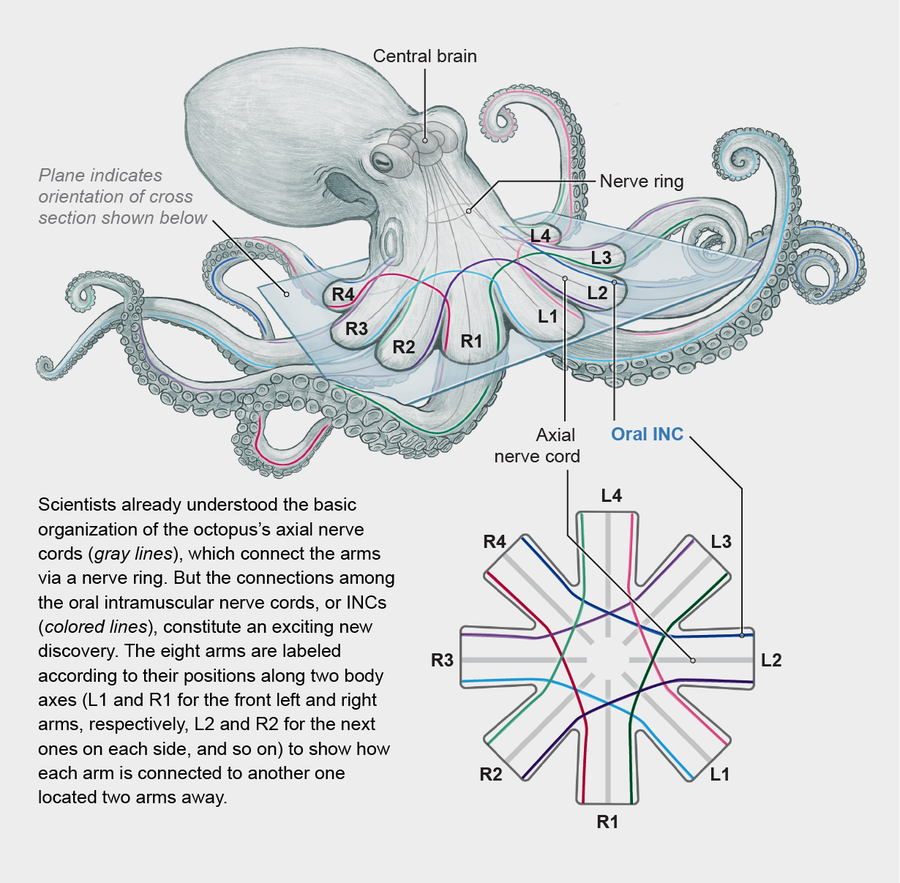
Diagram of the nervous system of an octopus. Source: “Multiple Nerve Cords Connect the Arms of Octopuses, Providing Alternative Paths for Inter-Arm Signaling,” by Adam Kuuspalu et al.
Octopi are also known to be able to taste food and sense chemicals with the suction cups on their tentacles. They have an incredibly complex sensory system to match their extraordinary nervous system. Their eyes also operate similarly to humans, using a sort of 'camera lens'-type structure to focus on parts of the optical image. They can even see in a variety of colors.
But why do they need such high-powered mental prowess? Researchers don't have a complete answer, but a large piece of the puzzle likely has to do with hunting and predation. Octopi require a big nervous system to hunt in the tide pools of beaches without drying up in low tide and to escape a hungry barracuda on the edge of a coral reef. They aren't the kind of animal that can make millions of babies and afford to lose a few; to survive until reproduction, an octopus has to use its brain.
Arthropods: Shrimp
I'm saving the best for last.
Chordates
Because there are so many advancements in Chordata, rather than have each subgroup within the tab for the phylum, I have opted to create seperate pages for each order, except for simple vertebrates, with are covered below.
Simple Chordates
The most rudimentary of modern-day vertebrates is called the lancelet.
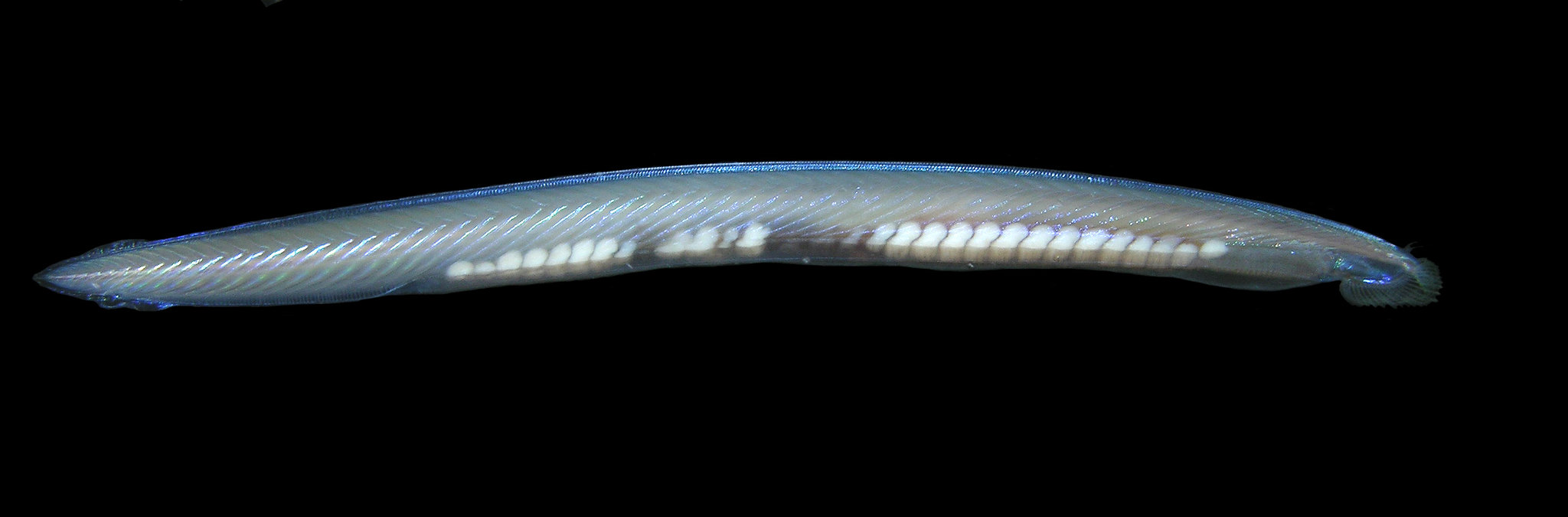
Stock image of a lancelet.
Lancelets and their relatives have no signs of cephalization, or the development of a brain. Instead, they have a nerve cord that branches off into segmented muscle regions of the body, not entirely unlike annelids. However, the feature that sets the nervous system of the lancelet apart for every other animal we've covered as of yet is their notocord.
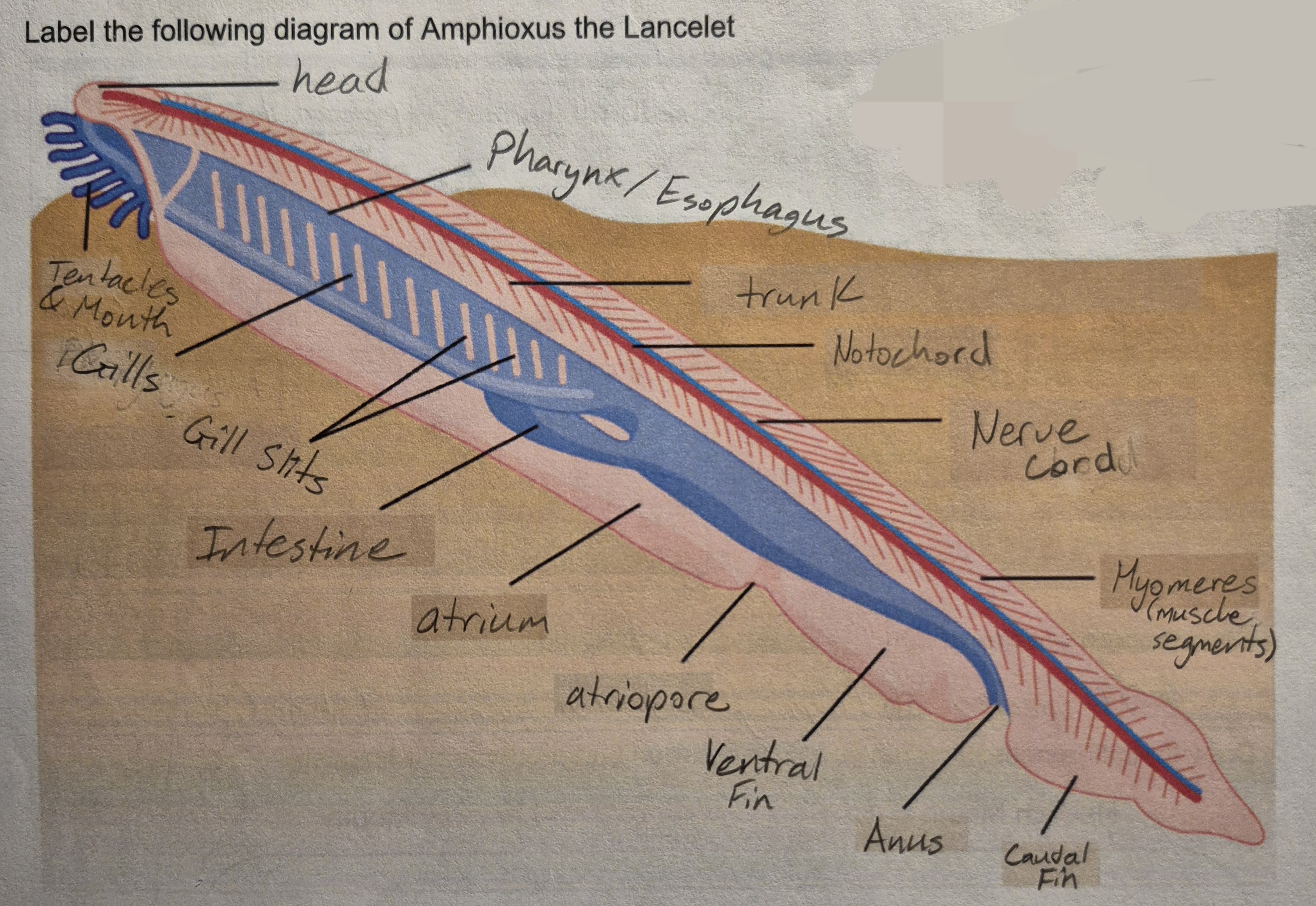
While the notocord is technically a part of the early vertebrate's skeletal structure and not the nervous system, its impact on the development of the nervous system was major. The notocord is equal to an early spinal cord, functioning to protect and support the nerve system. This combination of structure and nervous control was crucial to the evolution of more complex vertebrates.
For the lancelet personally, this advancement meant that the animal could swim with stronger, more precise movements. This allowed it to follow blooms of algae and colonies of microorganisms as they moved with the ocean's currents, keeping a filter-feeding lifestyle as an animal with almost hunter-like traits.